The Strong Force: Unraveling the Mysteries of Neutron Stars
Written on
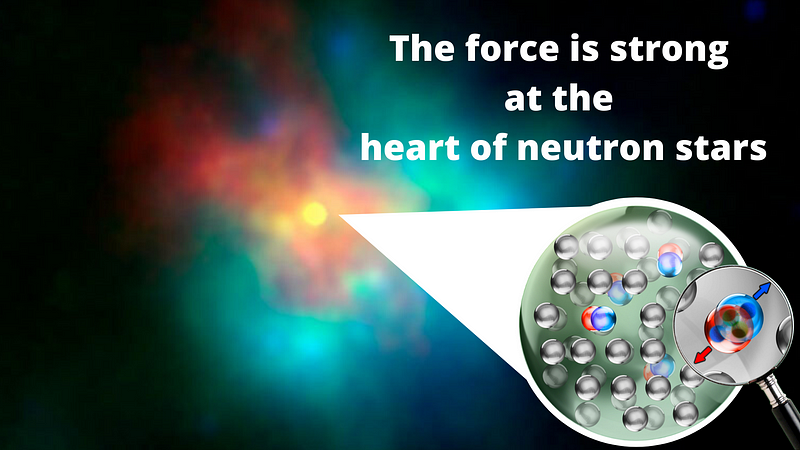
The strong nuclear force remains a fundamental yet elusive aspect of atomic structure, holding protons and neutrons together within atomic nuclei. This force is particularly challenging to study due to its short-range nature. A team of nuclear physicists, including researchers from the Massachusetts Institute of Technology (MIT) led by Or Hen, has developed an innovative approach to explore the strong force at extremely close distances, akin to conditions found in the cores of neutron stars.
“The research provides an unprecedented look at the strong nuclear force’s behavior at very short distances,” says Hen. “This has significant implications, especially for our understanding of neutron stars and nuclear systems in general.” Their findings, published in Nature, may initiate a new phase of experimental investigations into the strong force, potentially enhancing our comprehension of neutron star structures.
The universe's matter interactions are governed by four fundamental forces: gravity, electromagnetism, the weak nuclear force, and the strong nuclear force. While gravity and electromagnetism are more familiar to us, the latter two are equally vital. The strong nuclear force, for instance, is essential for the existence of matter as we know it.
alt: Searching for the strong force (MIT)
“The strong force binds the atomic nucleus—composed of protons and neutrons—at a distance of around 10^–15 m, while the electromagnetic force maintains the electron cloud at 10^-10 m,” explains Douglas Higinbotham, a professor of nuclear physics at Jefferson Labs. “At these short scales, the strength of the strong force far exceeds that of electromagnetism, and is vastly greater than gravity.”
Within the atomic nuclei that constitute everyday matter, protons and neutrons are sufficiently spaced apart for physicists to predict their interactions accurately. However, the situation complicates when these particles, known as nucleons, are in close proximity, as occurs in neutron stars and similar dense astrophysical objects. “The challenge lies in calculating the system since conducting experiments on neutron stars is impossible,” Higinbotham adds.
Historically, the investigation of the strong force at close distances has been hindered by complex mathematics. “While the governing equation of the strong force is straightforward, applying it for practical calculations can be tricky,” states Axel Schmidt, a co-author of the study. “Calculating interactions at high energies is relatively easy, but determining the force between closely positioned protons and neutrons poses significant challenges.”
Fortunately, a recent theoretical advancement allowed the team to undertake approximate calculations and reinterpret existing data from particle accelerators. “Instead of calculating the strong forces acting among all nucleons in a reaction, we focused on how a pair of nucleons in very close proximity could be influenced,” Schmidt explains.
To observe interactions between protons and neutrons at small distances, high-energy electrons must bombard atoms. A small fraction of these collisions can eject pairs of nucleons at high velocities, providing insights into their interactions. These collisions are only measurable at high-current particle accelerators, such as the JLab accelerator, which operated from 1988 to 2012.
alt: Jefferson Lab’s CEBAF Large Acceptance Spectrometer data collection.
The CLAS detector collected data from high-energy electron beams directed at various atomic nuclei. The MIT team uncovered new findings in data from a 2004 experiment, analyzing quadrillions of electron interactions with atomic nuclei.
“CLAS effectively measured and recorded particle trajectories emerging from collisions, which enabled us to conduct 'data mining' to explore questions unconsidered during the initial experiments,” Schmidt elaborates. When electrons collide with nucleons, the scattering energy correlates with the energy and momentum of the nucleon. Hen likens this to reconstructing the momentum of an object based on the force of a kick.
By isolating pairs of high-momentum nucleons, referred to as 'neutron star droplets,' and analyzing their momentum, the team inferred the distances between protons and neutrons. They found that as the distance decreases, an unexpected transition in their interactions occurs. “Previous neutron star models regarded protons and neutrons as independent, but experiments reveal that nucleons prefer to pair up,” Higinbotham notes.
alt: Using Electron Scattering Measurements to Test Nuclear Interaction.
The researchers categorized several hundred snapshots of nucleon interactions according to momentum. They observed a suppression of proton-proton pairs at lower momentum, suggesting that at short distances, the strong force predominantly pairs protons with neutrons. However, as they analyzed higher momentum interactions, more proton-proton and neutron-neutron pairings emerged, indicating that the strong force operates not only on proton-neutron pairs but also on like-particle pairs at short distances.
Schmidt elaborates, “We discovered that as protons draw closer, the attractive force transitions to a repulsive nature, indicating a 'repulsive core' in this interaction.” This clear detection of a repulsive core was unexpected and suggests that protons and neutrons can be treated as overlapping in interactions, while still being modeled as individual particles.
This hard repulsive core of the strong nuclear force has not been experimentally accessed within a nucleus before. Efforts to achieve short distance measurements in particle accelerators typically resulted in interference from other particles.
The research has profound implications for understanding neutron stars, which are made up of some of the densest matter outside black holes. Formed from the gravitational collapse of dying stars, neutron stars possess cores so dense that a teaspoon would weigh as much as humanity.
alt: A neutron star at the center of a supernova’s remains.
“One significant application of this work is elucidating the conditions within neutron star cores, where neutrons are packed even more densely than in atomic nuclei,” Schmidt notes. “A better understanding of the strong force at short distances can clarify the pressure, size, and cooling rates of neutron stars.”
Current theories suggest that quantum mechanics alone prevents neutron star cores from collapsing entirely. Hen has previously indicated that neutrons in the outer core primarily pair with protons through the strong force. This new research suggests that as particles are compressed into denser configurations, the strong force generates a repulsive effect among neutrons, potentially stabilizing the neutron star.
The findings align with existing models, notably the Argonne V18 model, which considers various nucleon interactions at short distances. This suggests that neutron star core calculations can utilize this model to estimate strong force interactions without delving into more intricate quark and gluon interactions, challenging prior assumptions about neutron stars.
Hen adds, “The cores of neutron stars might be simpler than previously thought, which is surprising.” Higinbotham concurs, stating, “The success of the AV18 nucleon-nucleon potential in extreme conditions raises intriguing questions about its underlying reasons.”
The implications of this research extend beyond neutron stars, contributing to nuclear physics as a whole. “A deeper understanding of the strong force is invaluable for nuclear physics, as many calculations rely on approximate models of nucleon interactions,” Schmidt explains.
“New experiments are poised to collect fresh data, which may transform our understanding of the strong force. CLAS has been succeeded by an enhanced detector, CLAS-12, and I am eager to begin experiments with it next year, anticipating a significant increase in collision data and improved neutron detection capabilities.”
“It’s an exciting time for this field as we look forward to the influx of new data!”
Special thanks to Axel Schmidt and Douglas Higinbotham.
Original research: https://www.nature.com/articles/s41586-020-2021-6