Exploring Quantum Boundaries: Advances in Quantum-Controlled Solids
Written on
Chapter 1: Quantum Control of Solids
Researchers are advancing the frontier between classical and quantum physics by successfully levitating and cooling glass nanobeads into a quantum state. This innovative approach involves laser-cooling a minuscule glass nanoparticle, which is significantly smaller than a grain of sand, into a quantum state. This breakthrough allows scientists to explore the ambiguous boundary that separates quantum mechanics from classical physics. Although the manipulated particle may be tiny by everyday standards, it possesses the density of a solid object and is vastly more complex than single atoms or molecules typically used to study quantum motion. The research team, composed of physicists from institutions such as the University of Vienna and MIT, published their findings in the journal Science.
Dr. Markus Aspelmeyer, a co-author and professor of quantum optics at the University of Vienna, explains, "In classical physics, we expect a solid to exhibit a distinct position and momentum. However, quantum mechanics introduces uncertainties in these observable properties, leading to exotic phenomena unique to this field." He elaborates on the concept of superposition, where objects can exist in multiple states simultaneously, referencing the famous Schrödinger's cat thought experiment, which illustrates how a cat can be both alive and dead at the same time. "This concept challenges our everyday understanding," he adds. "In a practical sense, objects can exist in superpositions of various positions, interacting with their environment from multiple locations at once."
Physicists have long recognized that the quantum characteristics of individual atoms can be manipulated using lasers. This level of control has even been demonstrated with clouds of hundreds of millions of atoms, prompting them to enter macroscopic quantum states, including quantum gases and Bose-Einstein condensates—states of matter where separate atoms, cooled to nearly absolute zero, behave as a single quantum entity. The current research extends these principles to solids, which have a density billions of times greater than gases, where atoms are collectively bound and move in unison across the object's center of mass.
"In our study, we achieved a quantum ground state by removing all thermal energy from the system," Aspelmeyer explains. "In classical terms, this would mean the object remains completely still, but quantum theory predicts a residual energy—the vacuum energy—that leads to fundamental uncertainties in position and momentum."
The first video, "Assembling and Controlling Photonic Quantum Matter: Solids, Fluids and Cats," explores how researchers manipulate quantum states and the implications of their findings in quantum technology.
Chapter 2: Innovative Techniques in Quantum Control
Aspelmeyer highlights a key distinction between their experiment and previous research involving quantum solids. Unlike earlier experiments that utilized anchored mechanical oscillators, such as small diving boards that flex up and down, their solid is levitated using an optical trap. "We can, in principle, rapidly alter the optical trap to provide a dynamic potential landscape for the particle's movement," he says. "This flexibility enables us to generate strong nonlinear features that traditional clamped oscillators cannot produce."
The foundational method was initially introduced by Nobel laureate Arthur Ashkin several decades ago, becoming a well-established technique for isolating atoms from their environments. The expectation is that such experiments may pave the way for exploring macroscopic quantum states involving significant mass. Similar to Bose-Einstein condensates, these states could prove highly advantageous in advancing quantum technology and quantum computing.
"This area of research will be crucial in the future as we seek to increase the mass of our objects and investigate the quantum effects of larger systems," Aspelmeyer remarks. "In a solid, all atoms are bound to move cohesively, which allows for the generation of quantum states where all atoms occupy the same position—a feat that is exceedingly challenging with atomic gases."
Achieving quantum control over such sizable macroscopic particles presents a formidable challenge. The team selected a glass nanoparticle containing roughly (10^{12}) atoms for their experiments. For context, a single grain of sand contains about (4.33 times 10^{18}) atoms!
To gain quantum control over this macroscopic object, the researchers first had to isolate it from environmental influences, effectively cooling it down to near absolute zero. This process involved removing nearly all thermal energy and utilizing an optical trap within a vacuum to confine the particle, but the challenge of cooling it to near -273°C remained considerable.
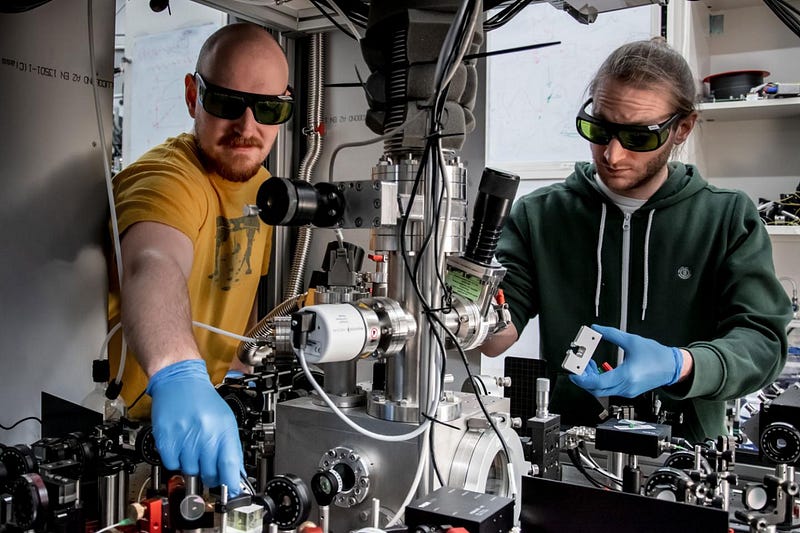
"The true challenge lies in cooling the particle's motion to its quantum ground state," says Uros Delic, lead author from the University of Vienna. "Laser cooling is well-established for atoms but has proven ineffective for solids." To overcome this limitation, the team developed a laser-cooling technique known as "cavity cooling by coherent scattering," originally proposed by Helmut Ritsch and studied by Vladan Vuletic and Nobel laureate Steven Chu. By improving this method—creating a purer vacuum and increasing photon input—the researchers successfully cooled the glass nanoparticle into the quantum regime.
Delic notes an intriguing paradox: "While the surface of our glass bead is extremely hot—around 300°C—due to the laser heating the electrons, the center of mass remains ultra-cold, just 0.00001°C above absolute zero. Thus, we can demonstrate that a hot particle is exhibiting quantum behavior."
"With the addition of cavity cooling by coherent scattering to our array of techniques, we have made significant progress after nearly a decade of research," Aspelmeyer adds. "The ability to modify the optical trapping potential provides a versatile means of altering the particle's quantum state."
The researchers are enthusiastic about the future implications of their work. "Optical levitation grants us much greater flexibility: by altering the optical trap—or even deactivating it—we can manipulate the nanoparticle's motion in entirely new ways," states Nikolai Kiesel, co-author and Assistant Professor at the University of Vienna. Several innovative schemes are now feasible, potentially leading to unprecedented advancements in sensing capabilities, the study of fundamental processes in quantum heat engines, and the exploration of quantum phenomena involving significant mass.
Dr. Aspelmeyer believes that the developments stemming from this research could ultimately assist physicists in addressing one of the most significant challenges in reconciling classical, relativistic, and quantum physics: the issue of quantum gravity. "A primary objective is to extend these experiments into the realm of large masses," he concludes. "In doing so, we may one day experimentally investigate the question of how a quantum system interacts with gravity."
The second video, "PHYSICS 295B: Quantum Theory of Solids: Lec 4. Electron gas," delves deeper into the theoretical aspects of quantum solids and their implications for future research in the field.
Original research: Deli, U., Reisenbauer, M., Dare, K., Grass, D., Vuleti, V., Kiesel, N., & Aspelmeyer, M. (2020). "Cooling of a Levitated Nanoparticle to the Motional Quantum Ground State," Science, DOI: 10.1126/science.aba3993. Special thanks to Markus Aspelmeyer and Kahan Dare.